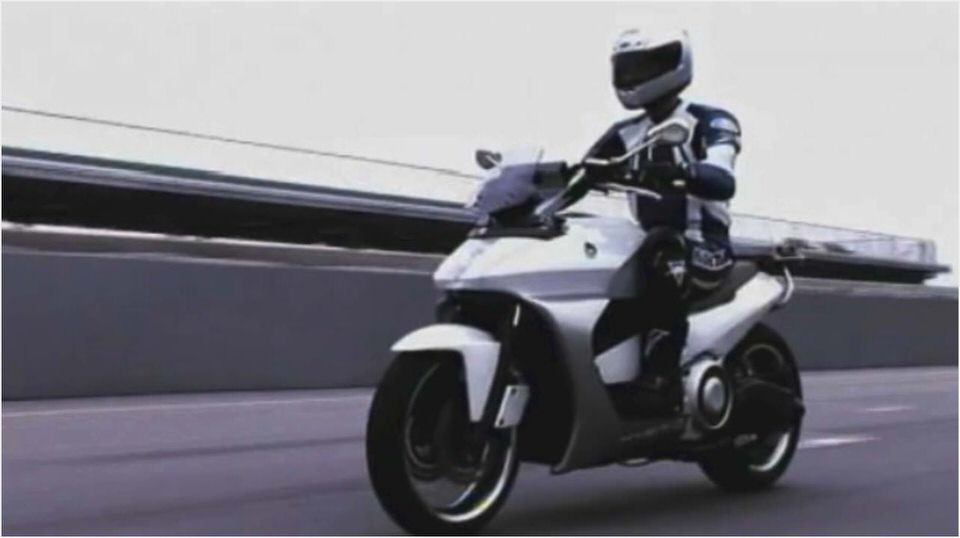
DoD 2013.3 SBIR Solicitation
SB133-001: Efficient Quantum Frequency Conversion for Advanced Optical Communications
OBJECTIVE: Conceive and develop methods and techniques for substantially improving the performance of optical signal processing in nonlinear optical devices. Of particular interest is developing technologies suitable for quantum information processing such as near-100%-efficient quantum frequency conversion.
Areas for development include achieving nonlinear processes with precisely tailored phase-matching spectra, reducing power requirements for driving such processes, developing single and few spatial-mode waveguides with ultralow level of background noise, reducing end-to-end loss, and robust packaging of devices with input and/or output fiber coupling. DESCRIPTION: Frequency conversion that preserves the quantum states of the converted signals, i.e. the so-calledquantum frequency conversion(QFC), has found numerous applications due to its ability to manipulate coherent or nonclassical state light.
For example, advanced optical communications applications, including single-photon quantum communications and quantum information processing for provably secure data links, can benefit from operation in both the visible/near- IR wavelengths (400800 nm) and the telecommunications C-band (15301565 nm). Visible and near-IR wavelengths enable coupling to atomic systems / quantum dots (e.g. for quantum memory) and detection via fast, high-efficiency, room-temperature single-photon detectors; but, unfortunately, visible/near-IR photons cannot be transmitted over long distances through single-mode fiber.
C-band photons can be transmitted over long distances with extremely low loss using the existing single-mode fiber telecommunications grid, but can be neither coupled to atomic systems nor easily detected. In principle, QFC provides the ideal solution: coherent transfer between visible photons (for photon processing, storage, and detection) and telecommunications photons (for long-haul transmission through fiber).
Perfecting this technology could bridge the distance gap that limits the state-of-the-art in provably secure communications, thus opening the door for a revolutionary capability for the DoD. Thus far, QFC has been demonstrated in various nonlinear optical media, such as crystals, waveguides, microresonators, and optical fibers. In such systems, quasi-phase matching (QPM) has been an enabling technology to allow efficient nonlinear interactions to be designed over a large wavelength span.
In nonlinear crystals or waveguides, QPM is usually achieved via periodic poling, whereas in nonlinear microresonators, it can be realized utilizing the modal dispersion of the cavity modes. While some applications of QPM have become commercialized, others are emerging that place more stringent requirements on device fabrication and processing. For example, QFC may prove to be important for a host of quantum information processing applications [1].
For some such applications it would be advantageous to obtain a narrow phase matching peak, enabling a potential direct interface to quantum memory, for example. On the other hand, a broad phase matching bandwidth is useful when up-converting a high-rate single-photon channel since it allows the channel to be time-multiplexed to multiple detectors by exploiting multi-wavelength pumps [2].
In addition to reaching higher speed performance, such a system allows high efficiency Si avalanche photodiode (APD) single-photon counters to be used at a wider variety of wavelengths, including the telecom bands where single-photon counters tend to have poor efficiency, limiting the reach of quantum communications. Optical up- conversion with a tunable laser followed by Si APDs can also be used to build an extremely sensitive spectrometer [3].
In such a case a narrow band single phase matching peak is highly desirable because a narrow band leads to higher resolution while a single peak reduces unwanted cross talk. Quantum applications are in general highly sensitive to very small (i.e. single photon) light leakage levels from scattering processes such as Raman scattering [4].
Moreover, quantum systems are particularly sensitive to loss but most waveguide based frequency converters have significant (3dB) loss especially when coupled to standard optical fibers. In addition to quantum applications, classical applications in instrumentation are also emerging such as measuring the jitter of attosecond optical pulses [5]. Such applications can benefit from waveguides that support dual polarizations and Type-II nonlinear interactions.
Type-II phase matching can also be employed for pre- screening waveguides prior to poling to improve yield and uniformity [6]. These emerging applications demand a new generation of nonlinear waveguides with different and more stringent performance metrics. Thus it is of great practical importance that these new waveguides can be made with high yield, so the cost is not a limiting factor.
Innovative solutions are sought to the problem of robust quantum frequency conversion. Successful solutions should simultaneously address all of the following critical technological challenges. (1) Low loss.
Nonlinear waveguides are limited by coupling loss (from single-mode fiber for the C-Band signal, to single-mode fiber or free space for the visible signal), insertion loss (to and from the waveguide itself) and transmission loss (per unit length) through the frequency conversion medium; the net loss from all sources limits device performance and it is this net loss that should be compared to program milestones. (2) Ability to tailor the phase-matching bandwidths as detailed above. (3) Wavelength selectability. A completely successful solution should provide the flexibility to couple any wavelength in the visible/near-IR to any wavelength in the C-band.
Note that this flexibility need not be in real time, but should instead reflect a flexibility in the fabrication process that allows any one device to be tailored for any two specific wavelengths. For high-energy photons near 400-nm, multiple stage frequency conversion may be required. (4) Efficiency. The waveguide nonlinearity should be sufficiently strong to provide near 100%-efficient coherent (entanglement-preserving) frequency conversion. (5) Robustness and portability.
The ultimate goal of this SBIR is to develop a packaged, robust, portable, room-temperature technology that can be inserted into an existing system. PHASE I: Develop a detailed computer model for the performance of a quantum frequency conversion technology which fulfills all of the above requirements.
Perform a detailed simulation and preliminary experimental evaluation of end- to-end device performance, and predict the technologys loss, noise, speed, wavelength flexibility, efficiency, and robustness under a variety of realistic conditions and for the target wavelengths (400800 nm and 15301565 nm). A plan for reducing end-to-end losses from all sources to1.5 dB should be identified. The impact of Raman photons shall be considered and methods to achieve low noise shall be identified.
Based on the simulation results, each team should make quantitative predictions about PHASE II and PHASE III device performance in each of the five categories listed above. Using these predictions, establish appropriate PHASE II technical milestones on a six-month (all milestones must include a quantitative target) incremental basis. PHASE II: Construct prototype devices whose end-to-end performance effectively demonstrates the contractor’s technical approach.
Using these devices, test all PHASE I predictions for device performance. In particular, singly peaked phase matching bandwidths of0.2 nm must be demonstrated. Losses and scattering effects must be minimized making the devices suitable for quantum applications, possibly those in a cascaded environment. Low end-to-end losses (1.5 dB) through the nonlinear material must be demonstrated. Methods to maintain acceptable yields must also be developed.
After characterizing the prototype devices, further refine and extend the PHASE I models. Finalize a design for a production system capable of delivering tailored devices as final products, and use the refined models to predict the performance of the second-generation (PHASE III) devices.
PHASE II deliverables will include device prototypes operating in multiple operational regimes (e.g. at different source and target wavelengths), intermediate reports quantifying progress towards fulfilling the proposed technical milestones, and a final report summarizing all simulations, models, device designs, quantitative predictions for PHASE III device performance, and proposed quantitative milestones for PHASE III. PHASE III: Secure optical communications are critical to both the DoD mission and to many commercial systems.
DARPA is currently funding a major effort to create macroscopic quantum communications systems capable of combining quantum security with classical telecommunications speeds and distances (The QUINESS Program). Deployment of this technology will require exactly the type of robust frequency conversion devices described in this SBIR.
More broadly, any sensing or communications system which requires detection of ultra-low levels of telecommunications-band radiation will benefit from a frequency conversion technology that enables use of superior visible-light single-photon detectors. The new breed of nonlinear frequency conversion is foreseen to be used in a variety applications including single photon detection for quantum information or lidar, and classical measurements such as low photon spectroscopy or highly accurate laser pulse jitter measurements.
SB133-002: Defense Against National Vulnerabilities in Public Data
This topic is eligible for the DARPA Direct to PHASE II Pilot Program. Please see section 7.0 of the DARPA instructions for additional information. To be eligible, you must submit documentation which demonstrates that Phase I feasibility (as described in PHASE I below). Offerors must choose between submitting a PHASE I proposal OR a Direct to Phase II proposal, and may not submit both for the same topic.
OBJECTIVE: Investigate the national security threat posed by public data available either for purchase or through open sources. Based on principles of data science, develop tools to characterize and assess the nature, persistence, and quality of the data. Develop tools for the rapid anonymization and de-anonymization of data sources.
Develop framework and tools to measure the national security impact of public data and to defend against the malicious use of public data against national interests. DESCRIPTION: The vulnerabilities to individuals from a data compromise are well known and documented now asidentity theft.These include regular stories published in the news and research journals documenting the loss of personally identifiable information by corporations and governments around the world.
Current trends in social media and commerce, with voluntary disclosure of personal information, create other potential vulnerabilities for individuals participating heavily in the digital world. The Netflix Challenge in 2009 was launched with the goal of creating better customer pick prediction algorithms for the movie service [1].
An unintended consequence of the Netflix Challenge was the discovery that it was possible to de-anonymize the entire contest data set with very little additional data. This de-anonymization led to a federal lawsuit and the cancellation of the sequel challenge [2]. The purpose of this topic is to understand the national level vulnerabilities that may be exploited through the use of public data available in the open or for purchase.
Could a modestly funded group deliver nation-state type effects using only public data? The threat of active data spills and breaches of corporate and government information systems are being addressed by many private, commercial, and government organizations. The purpose of this research is to investigate data sources that are readily available for any individual to purchase, mine, and exploit.
The marketing community uses large-scale data aggregators, big data analytics, and social science techniques to deliver highly targeted advertising campaigns. Does the availability of data for purchase or for free, advanced marketing techniques (e.g. collaborative filtering, computational advertising), and low-cost big data analytic capabilities (e.g. Amazon EC2) provide a determined adversary with the tools necessary to inflict nation-state level damage?
To what extent could a non-state actor collect, process, and analyze a portfolio of purchased and open source data to reconstruct an organizational profile, fiscal vulnerabilities, location of physical assets, work force pattern-of-life, and other information [3], in order to construct a deliberate attack on a specific capability? The goal of this topic is to develop tools to characterize and assess the nature, persistence, and quality of data.
The tools should be based on principled scientific methods for sampling and relevant statistical methods for assessment. Also of interest are tools to characterize the quality of data for automated processing and analysis (i.e. a measure of how much manpower would be required to use a specific source). Additionally, the goal of this topic is to characterize the threat through the creation of tools, techniques, and methodologies to measure the vulnerabilities in a given set of public data.
As an example, reconstructing the profile of an organization from many data pieces using low computational-complexity methods might indicate vulnerability. Also of interest to this topic is the development of sensors, tools, and techniques necessary to defend against the malicious use of data for purchase. Throughout the performance of this research (Phases I, II, and III), there will be no indefinite collection or storage of data sources containing personal identifying information (PII).
Develop a proof-of-concept system that can automatically sample data from numerous sources, characterize the data, and provide automatic feedback on the measurable risk inherent with various collections of data. Develop methodology for risk assessment and mitigation through reallocation of resources. PHASE I: Investigate the landscape of public data both open and purchasable across several domains (e.g.
GIS, webpages, consumer data, social media, etc.), through statistical data characterization and assessment. Develop a set of risk factors for vulnerability including complexity of the computation for compromise, and design a prototype tool set necessary to automatically measure the risk inherent in the data. Develop a plan for detailed implementation of methods in PHASE II and III, including a data privacy plan.
DIRECT TO PHASE II – Offerors interested in submitting a Direct to PHASE II proposal in response to this topic must provide documentation to substantiate that the scientific and technical merit and feasibility described in the PHASE I section of this topic has been met and describes the potential commercial applications. Documentation should include all relevant information including, but not limited to: technical reports, test data, prototype designs/models, and performance goals/results.
Read and follow Section 7.0 of the DARPA Instructions. PHASE II: Develop a proof-of-concept system that can automatically sample data from numerous sources, characterize the data, and provide automatic feedback on the measurable risk inherent with various collections of data. Develop methodology for risk assessment and mitigation through reallocation of resources.
PHASE III: DOD entities including Army, Navy, and Air Force are interested in operational security and not having their plans and operations compromised through vulnerabilities in public data. In addition, closing the any gaps in such vulnerabilities will minimize the attack front, in which the commercial organizations have interest. The goals for this Phase, aimed at developing capabilities for defensive countermeasures, are as follows.
Deploy a tool into a near-real-time environment that continually monitors available open source data, measures vulnerabilities, and provides defensive countermeasures. Develop a series of capabilities relevant to both government and commercial organizations to defend against threats due to the proliferation of purchasable or public data sets.
Deploy a tool into a near-real-time environment that continually monitors available open source data, measures vulnerabilities, and provides defensive countermeasures. Develop a series of capabilities relevant to both government and commercial organizations to defend against threats due to the proliferation of purchasable or public data sets.
This topic is eligible for the DARPA Direct to PHASE II Pilot Program. Please see section 7.0 of the DARPA instructions for additional information. To be eligible, you must submit documentation which demonstrates that PHASE I feasibility (as described in PHASE I below).
Offerors must choose between submitting a PHASE I proposal OR a Direct to PHASE II proposal, and may not submit both for the same topic. OBJECTIVE: Use measurable electronic and/or physical characteristics to identify the specific fabrication facility of origin of a given electronic component.
DESCRIPTION: DARPA seeks innovative experimental and theoretical research leading to the identification of specific process signatures on an electronic component which can be uniquely associated with the semiconductor fabrication facility that manufactured the component. Current methods of identifying fab of origin are generic.
These methods currently include matching observed on-chip ground rules used for polygon widths and spaces to values connected to a particular company in the literature; or finding distinctive structures or materials that are associated with specific company advertised capabilities. The Department of Defense anticipates multiple uses for such a capability, generally in the domain of improving supply chain integrity.
This project seeks to find specific structural, chemical, magnetic, or electrical properties of generic on-chip features which are unique to a semiconductor fabrication facility, and which can be used as fingerprints to deterministically identify the origin of a component. Even within the same lithography node and process gate definition, certain idiosyncrasies associated with manufacturing tool installations, altitude and geomagnetic location of the facility, and chemical sources may assert detectable differences in the resulting product which can be observed or measured.
Examples of facility-specific structure differences might include: specific sidewall etch angle, front-end-of line agglomeration, amount of gate activation, dose/energy/junction positioning of implants, segregation of dopants, gettering, metal line grain sizes, or wire liner thickness isotropies. Virtually thousands of characteristics and parametrics might be associated with a given chip, and indeed multiple chips and processes might be produced in a given fab simultaneously; from these, the performer will be expected to identify those parametrics that are sufficiently unique in their statistical presentation, even when compared to the same process installed in other semiconductor fabrication facilities, or across different processes.
It should be assumed that these features must be recognized without the benefit of having a specific metrology or reference structures pre-placed for the purpose of identification. Analysis shall be limited to features commonly available on electronic components. While non-destructive electrical testing to determine identity provides the most utility for the application, proposals advancing destructive analysis are also acceptable.
Techniques which in addition enable an estimation of the date of manufacture are encouraged. It is anticipated that in the execution of the project, a body of knowledge will be accumulated which characterizes multiple major semiconductor fabrication facilities worldwide. PHASE I: Develop a concept for identifying a semiconductor fabrication facility through the analysis of the electrical parameters of the components that are built at that facility.
Through experimentation, identify the parameters, or combination of parameters, that can be used to create a measurable, repeatable signature for purposes of identification. Determine the technical feasibility of measuring the identified parameters and using those measurements to uniquely identify the manufacturing origin of an electronic component.
DIRECT TO PHASE II – Offerors interested in submitting a Direct to PHASE II proposal in response to this topic must provide documentation to substantiate that the scientific and technical merit and feasibility described in the PHASE I section of this topic has been met and describes the potential commercial applications. Documentation should include all relevant information including, but not limited to: technical reports, test data, prototype designs/models, and performance goals/results.
Read and follow Section 7.0 of the DARPA Instructions. PHASE II: Develop, demonstrate and validate a method for electrical and/or physical characterization of an electronic component that can be used to identify the semiconductor fabrication facility where the component was manufactured.
To prove that the chosen identifying characteristics are unique to a specific manufacturing facility, validation should include the testing of parts acquired from several facilities, including testing multiple parts from each facility. The expectation is to be able to create a database of signatures which comprise the identifying characteristics for each semiconductor fabrication facility.
PHASE III: Potential military applications include hardware integrity assurance, anti-tampering, threat identification, supply chain risk management and failure analysis. The ability to identify the specific fabrication facility of origin of a given electronic component has utility in a number of uses, including supply chain risk management actions, intellectual property rights and licensing enforcement, component dating and vintage analysis, and inventory control. Commercial applications include the detection of counterfeit electronic parts, establishment of intellectual property rights and licensing enforcement, supply chain risk management, hardware failure analysis and inventory control.
SB133-004: Hybrid Off-Road Motorcycle
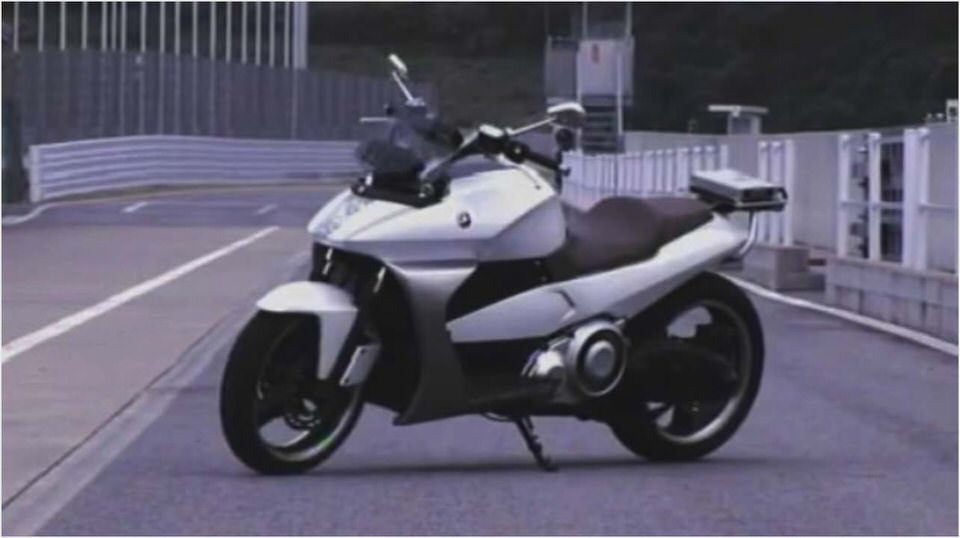
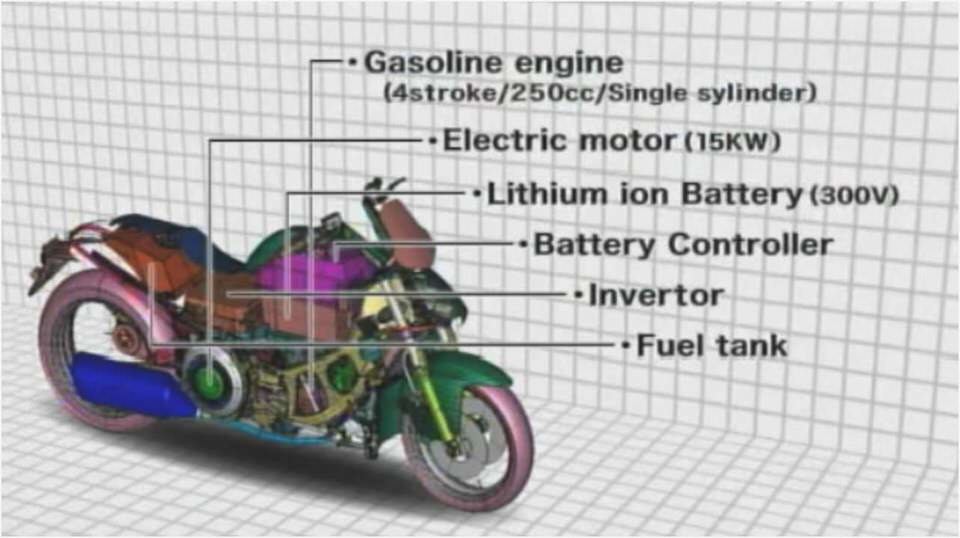
This topic is eligible for the DARPA Direct to Phase II Pilot Program. Please see section 7.0 of the DARPA instructions for additional information. To be eligible, you must submit documentation which demonstrates Phase I feasibility (as described in PHASE I below). Offerors must choose between submitting a Phase I proposal OR a Direct to Phase II proposal, and may not submit both for the same topic.
OBJECTIVE: Develop the required technologies and demonstrate a lightweight two-wheel drive (2WD) hybrid-electric off-road motorcycle for combat troop use powered by heavy fuels, capable of short periods electric-only propulsion, and usable as a portable electric power source for soldiers in the field. DESCRIPTION: This topic is based on four principal motivating factors: (1) Mobility for deployed forces is enhanced by reducing the logistical support requirements for volatile fuels (e.g. gasoline) and utilization of readily-available heavy fuels (e.g. diesel, JP-8) for vehicles and electrical power generation and by reducing the need to carry batteries for electric/electronic equipment. (2) Mobility through harsh unimproved terrain is difficult due to soft soils, narrow, and steep trails.
A lightweight approach to effective two-wheel drive in motorcycles is highly desirable. (3) A rapid and stealthy approach toward enemy combatants enhances the element of surprise, but the vehicles used for rapid mobility are generally compromised by vehicular noise. (4) A desire to improve vehicular fuel efficiency. Technologies relevant to this SBIR are being pursued for a variety of mobility applications.
Higher power density diesel engines are being developed for aviation, although these tend to be of a larger capacity than desired for motorcycles. While smaller than aviation diesels, auto diesels are typically twice the weight of their equivalent gasoline-fueled counterparts. The US Military had purchased the Hayes M1030M1 diesel-powered motorcycle, but that vehicle is no longer in production.
The Dutch EVA Trac T800 is the only heavy-fuel motorcycle currently on the market and it is designed around a purpose-built diesel engine. Heavy fuel fed reformers and fuel cells have been used for stationary power applications. They are starting to see limited market penetration in larger mobility applications. The DARPA Vulture program for example is pursuing fuel cells with an energy density suitable for aircraft applications.
Turbines have been used for their heavy fuel capability by a variety of platforms, ranging from tanks to missiles. Unfortunately, they often suffer from comparatively high fuel consumption and noise suppression challenges. Hybrid-electric power has penetrated a wide variety of mobility markets ranging from construction equipment and busses to personal automobiles.
Following over 15 years of exploratory work by universities, small start-ups, and major motorcycle companies, hybrid motorcycles are on the verge of reaching the marketplace. A variety of hybrid concepts have appeared at shows including Yamahas Gen-Ryu and HV-X, and the Schneider-OCC hybrid electric chopper. The Piaggio MP3 Hybrid 300ie three wheeled scooter reached the market in 2010.
These examples, those that have tried and failed, as well as those that are currently under development have been for on-road applications, which can more easily tolerate greater weights. They have also relied on gasoline (Otto-cycle)/generator pairs or in a few instances, hydrogen fuel-cells. All-wheel drive or two-wheel drive motorcycles have been experimented with for nearly a century and have experienced limited commercial success.
The long-term American product is the small Rokon Ranger with a mechanically driven front wheel. Newer to the market is Christini Corps AWD 450. Other current developments include the hydraulically driven Yamaha WR450F 2-Trac, and KTMs recent patent for an electric front wheel drive.
While the various pieces of interest exist in isolation, nobody has successfully combined heavy fuel capability, 2WD, and hybrid powertrains into a useful off-road motorcycle. This is a very challenging component and system design problem. This SBIR proposes to address the challenge through the innovative application of technology to develop a motorcycle capable of improving support for soldiers operating in remote and harsh environments.
Desired characteristics of the vehicle include: Silent electric only mode and low noise (75 dB) during normal operation Production of supplemental power, e.g. for battery charging Two-wheel-drive in support of extreme terrain operations Heavy fuel compatibility10% improvement in load-specific fuel consumption as compared to existing fielded motorcycles PHASE I: Develop a preliminary design for the hybrid motorcycle, establish and validate performance goals, and develop a detailed analysis of expected performance. Benchmark expected performance against extant non-hybrid systems.
Demonstrate key elements of the hybrid propulsion and energy generation system, such as the engine, generator/alternator, motor, and power control. Deliver a report documenting PHASE I accomplishments.
DIRECT TO PHASE II – Offerors interested in submitting a Direct to PHASE II proposal in response to this topic must provide documentation to substantiate that the scientific and technical merit and feasibility described in the PHASE I section of this topic has been met and describes the potential commercial applications. Documentation should include all relevant information including, but not limited to: technical reports, test data, prototype designs/models, and performance goals/results.
Read and follow Section 7.0 of the DARPA Instructions. PHASE II: Further develop the concepts resulting from PHASE I into a complete, detailed, executable design that also addresses operational suitability, safety, manufacturability, maintainability, and operational durability. Produce a prototype motorcycle based on the developed design. Demonstrate the vehicle in operationally representative environments to verify and validate all functions and limitations of the design.
Prior to conducting human factors testing, develop and obtain approval for a human use plan. PHASE II deliverables include an operational prototype vehicle and a PHASE II report. PHASE III: The military could be expected to use this vehicle to replace motorcycles such as the M1030M1 currently in service.
Marine and Special Forces users will be particularly interested in enhanced mobility and silent running capabilities. Production versions of the resultant hybrid motorcycle would be expected to be purchased by Army and Marine units. Based on public interest in diesel and electric motorcycles, a substantial commercial market is anticipated.
This topic is eligible for the DARPA Direct to PHASE II Pilot Program. Please see section 7.0 of the DARPA instructions for additional information. To be eligible, you must submit documentation which demonstrates that PHASE I feasibility (as described in PHASE I below).
Offerors must choose between submitting a PHASE I proposal OR a Direct to Phase II proposal, and may not submit both for the same topic. OBJECTIVE: Develop robust manufacturing and strength improvement concepts for 2D laminate hot load bearing carbon-carbon structures. DESCRIPTION: The aerospace community has recently been successful in making complex large scale, hot structure (3000 deg), carbon-carbon (C-C) assemblies for high speed aerospace vehicles.
Future vehicle designs will push the limits of C-C material capabilities requiring a continued effort to characterize and improve the performance, robustness, availability, and affordability of integrated airframe structures fabricated using this material. Large scale assemblies drive the current state of the art due to the unique set of challenges they present, in particular thick aerodynamic and thermal load carrying laminates.
Technology development is required to produce stronger, more reliable, and more producible thick parts (reduced manufacturing time and cost) and thermo- mechanical stress models, as well as higher resolution and more informative non-destructive evaluations. Once developed, the structural properties and techniques will have an immediate impact on production time and vehicle weights, which will translate into quicker lead times and turnaround for multiple vehicle flight test programs.
The purpose of this SBIR is to improve the strength and consistency of thick C-C laminates used on large scale, load bearing airframe structures. With strength properties as the focus of improvements, improved properties will give more analytical confidence during the design phase of programs. The manufacturing techniques developed in the proposed program can also immediately be incorporated on current programs to increase margins in the most critical load areas.
The offeror must demonstrate a clear understanding of carbon-carbon processing technology of hot structures as applied to flight testing of hypersonic systems. Coinciding with improved strength properties, developing methods to rapidly make the thicker parts and confirm quality assurance are priority in the proposal.
PHASE I: Evaluate the in-process stress states of thick Carbon-Carbon laminates in order to optimize their fabrication, leading to improved strength properties and faster production. In parallel, identify and assess innovative non-destructive methods for evaluation of Carbon-Carbon laminates and structures to be employed in subsequent work to characterize and assure the quality of the laminates and the subcomponent structures.
Identify and evaluate existing and developmental material systems (e.g. fabric, heat treatment, resins, etc.) and identify and assess new methods as well as improvements to existing methods of creating thick C-C laminates in order to increase the mechanical properties and decrease the fabrication time. Prioritize and rank these methods and provide plans to develop and demonstrate those improvements. Conduct and report testing as needed to support these assessments.
The PHASE I deliverables will include monthly status reports and a final report with detailed process descriptions and material properties predictions supported by test demonstrations. DIRECT TO PHASE II – Offerors interested in submitting a Direct to PHASE II proposal in response to this topic must provide documentation to substantiate that the scientific and technical merit and feasibility described in the PHASE I section of this topic has been met and describes the potential commercial applications.
Documentation should include all relevant information including, but not limited to: technical reports, test data, prototype designs/models, and performance goals/results. Read and follow Section 7.0 of the DARPA Instructions. PHASE II: Develop and demonstrate new methods and improvements to existing methods of creating thick C-C laminates in order to increase the mechanical properties and decrease the fabrication time.
The bulk of the program and corresponding analysis requirements will involve the fabrication and testing of baseline material systems and the comparison to multiple experimental material systems that vary processing techniques, resins, processing schedules, and material. All of the variations will focus on accommodating the processing stresses by tailoring the properties of the material and distributing the loading.
Fabricate and conduct analysis and testing to characterize, relevant large scale C-C assemblies, including components with varying thickness and shape to demonstrate the processing approach viability and the predicted material properties. Deliverables for this Phase will be monthly status reports as well as a final report. Demonstrate and employ innovative non-destructive methods for evaluation of Carbon-Carbon laminates and structures to characterize and assure the quality of the laminates and the subcomponent structures.
PHASE III: This technology is applicable to all branches of the DoD and Military. Key military applications may include, but are not limited to, hypersonic missiles, hypersonic ISR aircraft, or on-demand space access vehicle. This technology also has application in the commercial sector in the area of efficient commercial access to space.
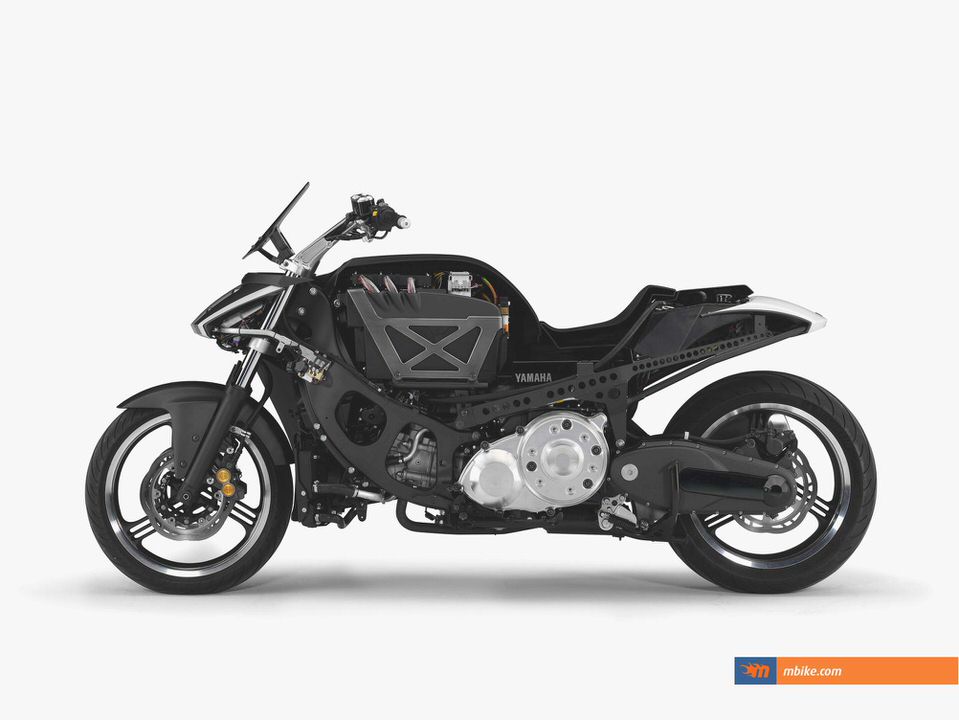
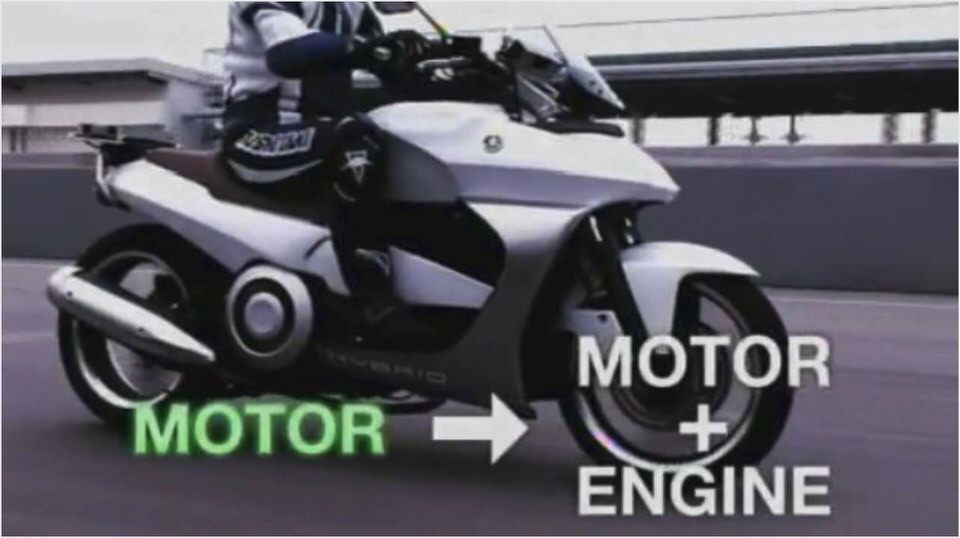
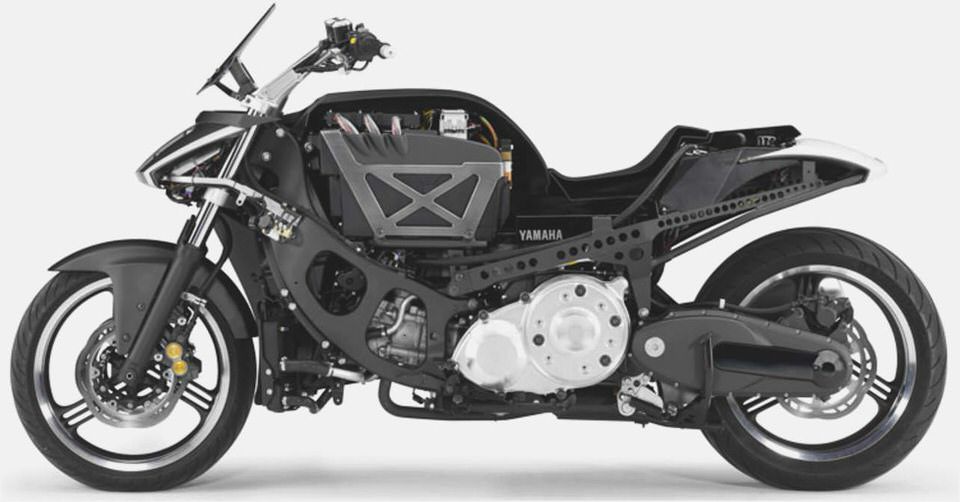
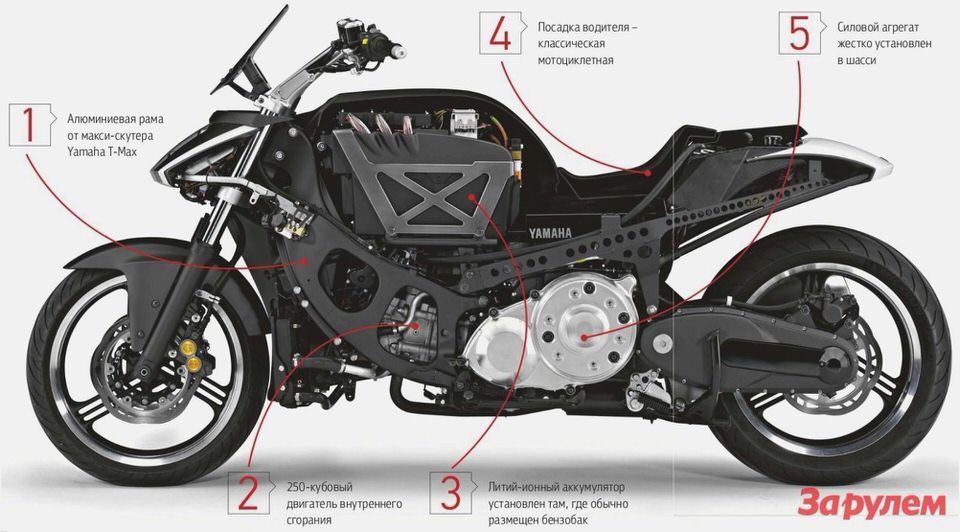
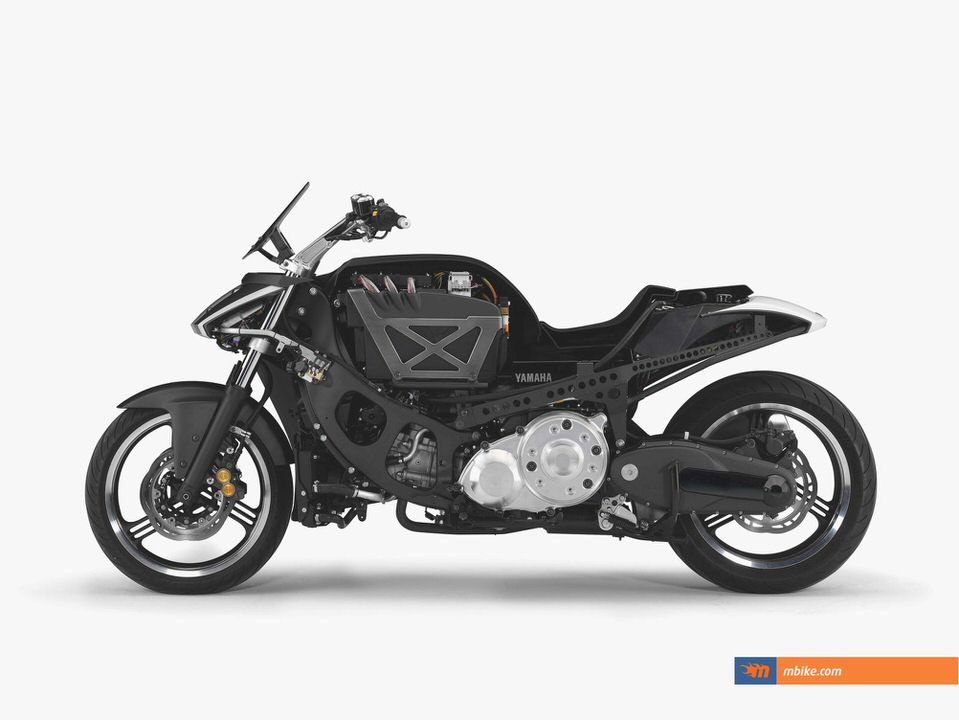
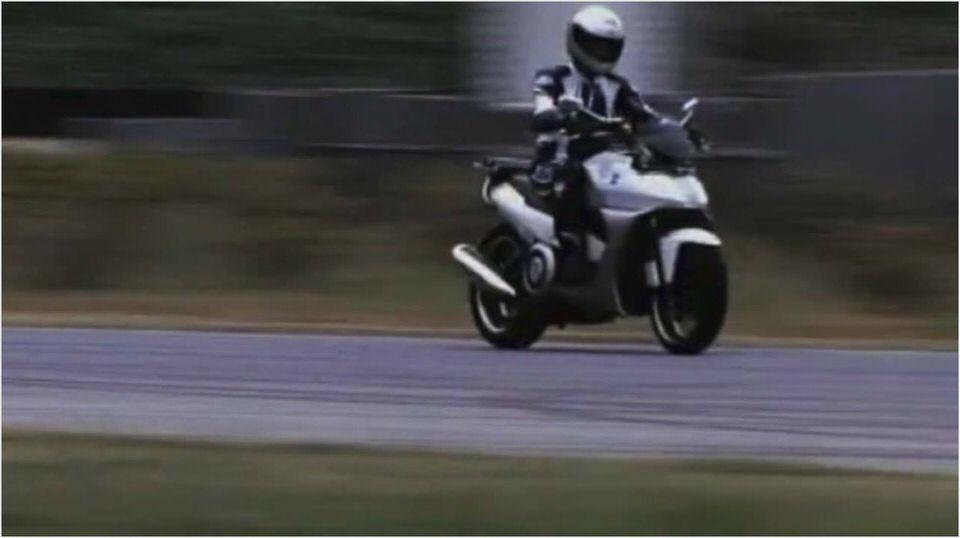
- Yamaha X-MAX 125 Sport 2011 Scooters Mopeds
- Yamaha YZR-M1 – Wikipedia, the free encyclopedia
- 2012 Yamaha XJR 1300 New Bikes Info
- YAMAHA STAR ROADLINER
- Customised Yamaha XV 1900 – Bike Reviews & News – Bikesales Mobi